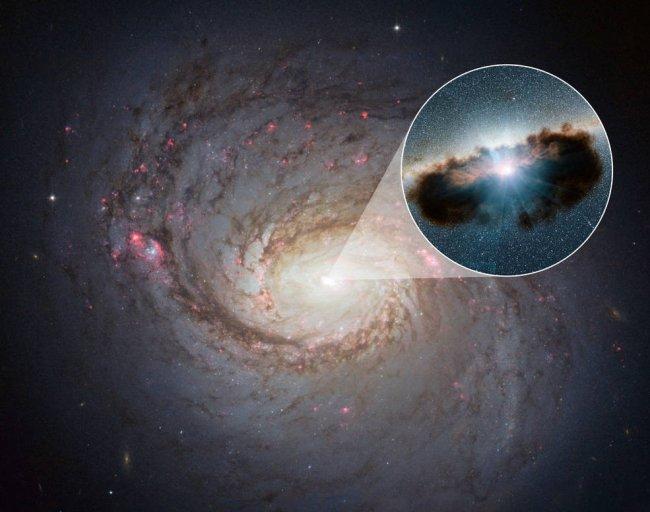
IMAGE: NASA Hubble Space Telescope image of Galaxy NGC 1068 with its active black hole shown as an illustration in the zoomed-in inset. A new model suggests that the corona around…
view more
Credit: NASA/JPL-Caltech
The origin of high-energy cosmic neutrinos observed by the IceCube Neutrino Observatory, whose detector is buried deep in the Antarctic ice, is an enigma that has perplexed physicists and astronomers. A new model could help explain the unexpectedly large flux of some of these neutrinos inferred by recent neutrino and gamma-ray data. A paper by Penn State researchers describing the model, which points to the supermassive black holes found at the cores of active galaxies as the sources of these mysterious neutrinos, appears June 30, 2020 in the journal Physical Review Letters.
“Neutrinos are subatomic particles so tiny that their mass is nearly zero and they rarely interact with other matter,” said Kohta Murase, assistant professor of physics and of astronomy and astrophysics at Penn State and a member of Center for Multimessenger Astrophysics in the Institute for Gravitation and the Cosmos (IGC), who led the research. “High-energy cosmic neutrinos are created by energetic cosmic-ray accelerators in the universe, which may be extreme astrophysical objects such as black holes and neutron stars. They must be accompanied by gamma rays or electromagnetic waves at lower energies, and even sometimes gravitational waves. So, we expect the levels of these various `cosmic messengers’ that we observe to be related. Interestingly, the IceCube data have indicated an excess emission of neutrinos with energies below 100 teraelectron volt (TeV), compared to the level of corresponding high-energy gamma rays seen by the Fermi Gamma-ray Space Telescope.”
Scientists combine information from all of these cosmic messengers to learn about events in the universe and to reconstruct its evolution in the burgeoning field of “multimessenger astrophysics.” For extreme cosmic events, like massive stellar explosions and jets from supermassive black holes, that create neutrinos, this approach has helped astronomers pinpoint the distant sources and each additional messenger provides additional clues about the details of the phenomena.
For cosmic neutrinos above 100 TeV, previous research by the Penn State group showed that it is possible to have concordance with high-energy gamma rays and ultra-high-energy cosmic rays which fits with a multimessenger picture. However, there is growing evidence for an excess of neutrinos below 100 TeV, which cannot simply be explained. Very recently, the IceCube Neutrino Observatory reported another excess of high-energy neutrinos in the direction of one of the brightest active galaxies, known as NGC 1068, in the northern sky.
“We know that the sources of high-energy neutrinos must also create gamma rays, so the question is: Where are these missing gamma rays?” said Murase. “The sources are somehow hidden from our view in high-energy gamma rays, and the energy budget of neutrinos released into the universe is surprisingly large. The best candidates for this type of source have dense environments, where gamma rays would be blocked by their interactions with radiation and matter but neutrinos can readily escape. Our new model shows that supermassive black hole systems are promising sites and the model can explain the neutrinos below 100 TeV with modest energetics requirements.”
The new model suggests that the corona–the aura of superhot plasma that surrounds stars and other celestial bodies–around supermassive black holes found at the core of galaxies, could be such a source. Analogous to the corona seen in a picture of the Sun during a solar eclipse, astrophysicists believe that black holes have a corona above the rotating disk of material, known as an accretion disk, that forms around the black hole through its gravitational influence. This corona is extremely hot (with a temperature of about one billion degrees kelvin), magnetized, and turbulent. In this environment, particles can be accelerated, which leads to particle collisions that would create neutrinos and gamma rays, but the environment is dense enough to prevent the escape of high-energy gamma rays.
“The model also predicts electromagnetic counterparts of the neutrino sources in `soft’ gamma-rays instead of high-energy gamma rays,” said Murase. “High-energy gamma rays would be blocked but this is not the end of the story. They would eventually be cascaded down to lower energies and released as `soft’ gamma rays in the megaelectron volt range, but most of the existing gamma-ray detectors, like the Fermi Gamma-ray Space Telescope, are not tuned to detect them.”
There are projects under development that are designed specifically to explore such soft gamma-ray emission from space. Furthermore, upcoming and next-generation neutrino detectors, KM3Net in the Mediterranean Sea and IceCube-Gen2 in Antarctica will be more sensitive to the sources. The promising targets include NGC 1068 in the northern sky, for which the excess neutrino emission was reported, and several of the brightest active galaxies in the southern sky.
“These new gamma-ray and neutrino detectors will enable deeper searches for multimessenger emission from supermassive black hole coronae,” said Murase. “This will make it possible to critically examine if these sources are responsible for the large flux of mid-energy level neutrinos observed by IceCube as our model predicts.”
###
In addition to Murase, the research team at Penn State includes the former IGC fellow Shigeo S. Kimura and Eberly Chair Professor Emeritus Peter Mészáros. The work was funded by the Alfred P. Sloan Foundation, the U.S. National Science Foundation, the Japanese Society for the Promotion of Science, NASA, the Penn State Institute for Gravitation and the Cosmos, and the Eberly Foundation.
TDnews